Glycolysis: fate of pyruvate under anaerobic and anaerobic conditions
You are well aware of the Glycolytic cycle/ glycolysis. It involves the splitting of glucose (glycol: sugar and lysis: split) to give a final product: pyruvate. Depending on the presence and absence of oxygen, the pyruvate has two fates. Let’s look at it in detail.
Once the glucose converts to in pyruvate, it further metabolizes. Pyruvate has three fates. Let’s look at each of them.
Case 1: In Aerobic organisms or tissues, pyruvate is oxidized in the presence of oxygen. In this process, it loses its carboxyl group as CO2 to give the acetyl group of acetyl Coenzyme A. The acetyl group is completely oxidized to CO2 by the citric acid cycle. The electrons from these oxidations will be passed on to O2 to form water. The energy from these electron transfer reactions will drive the synthesis of ATP. This is what happens in most humans.
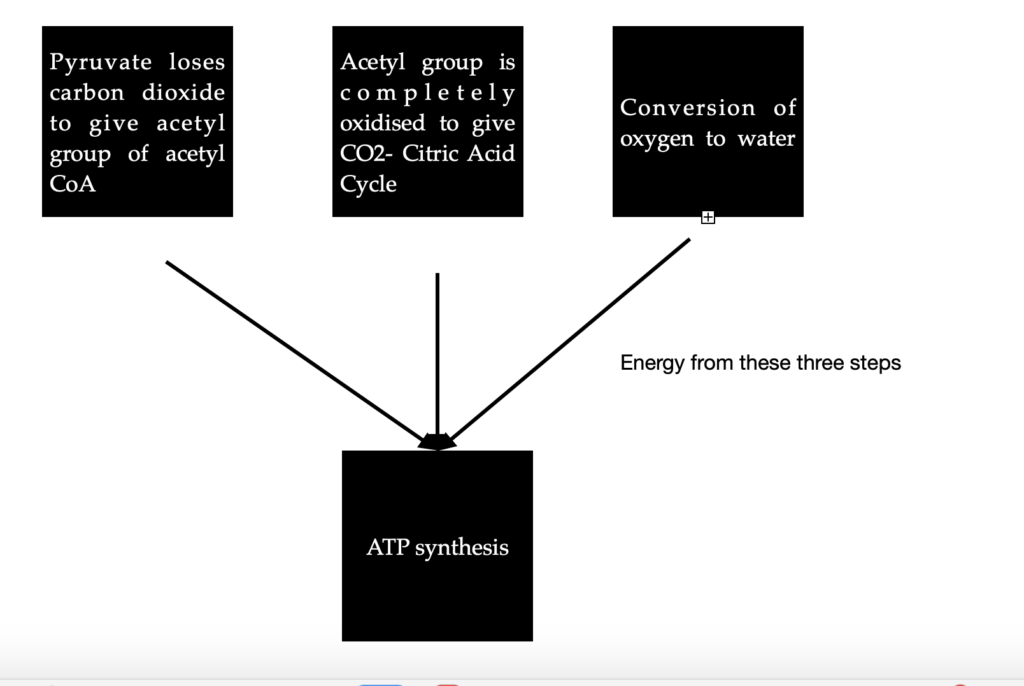
Case 2: In the second route, pyruvate is reduced to lactate through lactic acid fermentation. This condition, also known as hypoxia arises due to vigorous exercise. NADH cannot be re-oxidised to NAD+. But, we need NAD+ as an electron acceptor to further oxidize pyruvate. Due to the absence of its electron acceptor, pyruvate is reduced to lactate by accepting electrons from NADH. This step will generate the necessary NAD+ for the glycolysis to continue. Exception: some tissues and cells in our body convert glucose to lactic acid even under anaerobic conditions. They are red blood cells (erythrocytes) and retina.
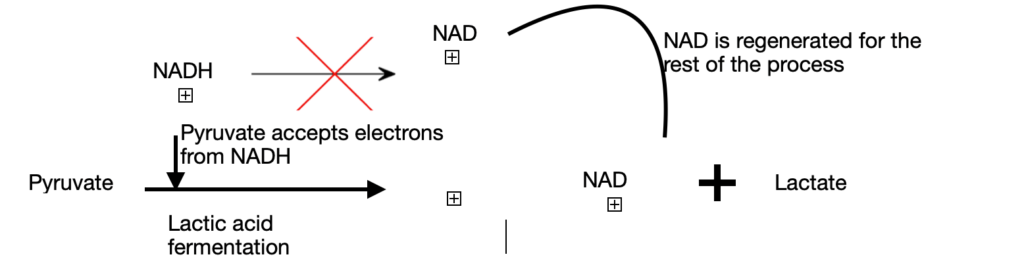
Case 3: the third route involves the conversion of pyruvate to ethanol. This is often seen in certain plant tissues, invertebrates like protists and microbes, etc. Like case 2, this process also happens in the absence of oxygen (anaerobic condition) to give ethanol and CO2. This process is called ethanol/ alcohol fermentation.
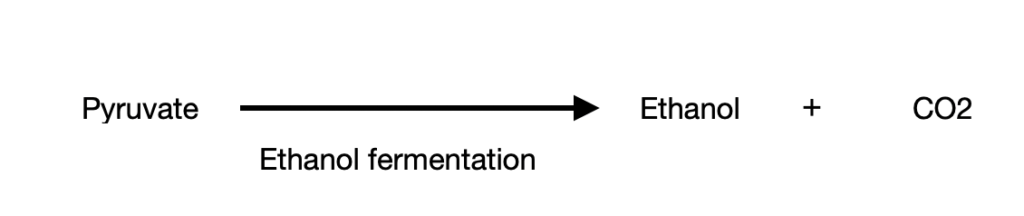
Glycogen synthesis
The storage form of glucose in most animals is glycogen. The synthesis of glycogen takes place in the liver and skeletal muscle.
- Glycogen synthesis starts from glucose 6 phosphate
- Upon undergoing a phosphoglucomutase reaction, it is converted to glucose 1 phosphate along with Uridine Tri Phosphate (UTP)
- Glucose 1 phosphate is acted upon by UDP-glucose pyrophosphate to give UDP-glucose + PPi
- UDP-glucose becomes the primary donor of glucose residues and donates glucose residues to the non-reducing end of glycogen. This reaction is catalysed by glycogen synthase.
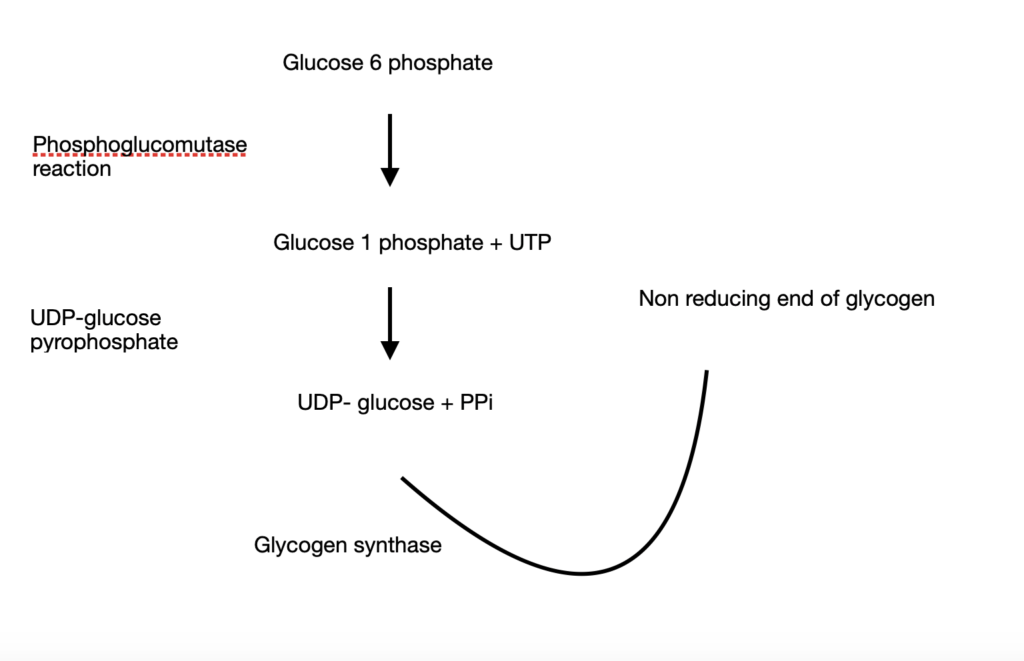
However, as you know, glycogen is a branched structure. Glycogen synthase catalyzes only the synthesis of linear chains of glycogen. The branched chain synthesis requires a special branching enzyme called amylo (1-4)(1-6) transglycolase.
Glycogen metabolism
Refer to my previous blog post to read more on glycogen metabolism.
Pentose phosphate pathway
Yet another important pathway in carbohydrate metabolism is the pentose phosphate pathway. It occurs in two phases: the oxidative phase and the non-oxidative phase. Let’s look at them in detail.
Oxidative phase
- Glucose 6-phosphate is converted to 6-phosphoglucono δ lactone along with NADPH with the help of the enzyme Glucose-6-phosphate dehydrogenase (G6PD). NAD+ is an electron acceptor
- The resulting lactone is hydrolyzed to 6-phosphogluconate with the help of lactonase
- 6-phosphogluconate, upon oxidation and decarboxylation by 6-phosphogluconate dehydrogenase will give Ribulose-5-phosphate along with NADPH
- Ribulose-5-phosphate undergoes isomerization to Ribose-5-phosphate
The overall reaction can be written as
Glucose 6 Phosphate + 2 NADP+ + H2O= Ribose 5 phosphate + CO2 + 2 NADPH + 2 H+
Ribose 5 phosphate is a precursor of nucleotide synthesis and NADPH is a reductant
In short,
Step | Substrate | Enzyme | Process | Product |
1 | Glucose 6 phosphate | Glucose 6 phosphate dehydrogenase | Dehydrogenation | Glucose 6 phospho glucono δ lactone |
2 | Glucose 6 phospho glucono δ lactone | Lactonase | Hydrolysed | 6 phosphogluconate |
3 | 6 phosphogluconate | 6 phosphogluconate dehydrogenase | Dehydrogenation | Ribulose 5 phosphate |
4 | Ribulose 5 phosphate | Isomerization | Ribose 5 phosphate |
In most organisms, the pentose phosphate pathway ends here. However, some tissues primarily require NADPH. In this case, it goes through another phase of the pentose phosphate pathway called the non-oxidative phase.
Non oxidative phase
- Ribulose 5 phosphate epimerizes to Xylulose 5 phosphate
- Upon rearrangements of the carbon skeleton of Xylulose 5 phosphate, six 5-carbon sugar phosphates are converted to five 6-carbon sugar phosphates thereby completing the cycle
- Upon continued recycling, glucose is eventually converted to 6 CO2 molecules. This step requires 2 enzymes: a transketolase along with the cofactor thiamine pyrophosphate (TPP) and transaldolase
Significance of pentose phosphate pathways
The PPP is an important pathway for several reasons including:
- Maintain carbon homeostasis
- Provide precursors for amino acid and nucleotide synthesis. One such precursor is ribose 5 phosphate, the final product of the oxidative phase of PPP
- Provide reductants like NADPH for anabolism
- Defeat oxidative stress
TCA cycle:
Also known as the citric acid cycle, it can be seen as a continuation of the glycolysis cycle. This is an important cycle and a central pathway for recovering energy from metabolic fuels like carbohydrates, fatty acids, and amino acids. The above fuel sources are first broken down to acetyl CoA for oxidation before entering the TCA cycle.
This cycle consists of a set of 8 reactions and by the end of the cycle produces two CO2, three NADH, one FADH2, and one high energy compound (GTP or ATP)
Net reaction: 3NAD+ +FAD+GDP+Pi +acetyl-CoA→ 3NADH+FADH2 +GTP+CoA+2CO2
Formation of acetyl CoA:
The first step in the process, even before the cycle starts is the synthesis of acetyl-CoA. It can be produced from amino acids or fatty acids, but, here we focus on carbohydrates.
- The end product of glycolysis under anaerobic conditions is lactate or ethanol. But, under aerobic conditions the final product is pyruvate.
- The pyruvate is converted to acetyl CoA through a multi-enzyme complex called pyruvate dehydrogenase. This enzyme complex contains three enzymes:
- Pyruvate dehydrogenase (E1)
- Dihydrolipolyl transacetylase (E2)
- Dihydrolipolyl dehydrogenase (E3)
They require 5 coenzymes including thiamine pyrophosphate, lipoamide, coenzyme A, and NAD+
Pyruvate, upon oxidative decarboxylation, converts to acetyl CoA.
- The pyruvate dehydrogenase complex catalyzes 5 reactions. Let us see each reaction in detail:
- E1 decarboxylates pyruvate with the formation of hydroxyethyl-TPP intermediate. It requires thiamine pyrophosphate (TPP) as a coenzyme.
- The hydroxyethyl group from the intermediate is transferred to the next enzyme E2 containing a lipoamide group. The hydroxyethyl group attacks the lipoamide disulfide, thereby eliminating TPP. This regenerates the active E1. While lipoamide reduces, the hydroxyethyl is oxidized to an acetyl group
- E2 transfers an acetyl group to Coenzyme A through a transesterification reaction. This yields acetyl CoA and dihydrolipoamide-E2
- Now that acetyl CoA has been formed, the lipoamide group of E2 must be regenerated. E3 reoxidizes to complete the catalytic cycle of E2. Oxidized E3 contains a Cys-Cys disulfide group and an FAD
- The reduced E3 is reoxidised. This step also converts NAD+ to NADH
The actual citric acid cycle starts after this step. Let’s look at the 8 reactions that make up the cycle.
Substrate | Enzyme | What it does | What it needs | Product | Intermediate |
Oxaloacetate and acetyl CoA | Citrate synthase | Condensation | H2O | Citrate | Citryl CoA |
Citrate | Aconitase | Isomerization | Isocitrate | Cis- Aconitate | |
Isocitrate | Isocitrate dehydrogenase | Oxidative decarboxylation | CoASH, NAD+, Mn2+ or Mg2+ cofactor | Alpha-keto glutarate + first CO2 and NADH | Oxalosuccinate |
Alpha-ketoglutarate | Alpha-ketoglutarate dehydrogenase | oxidative decarboxylation | CoASH, NAD+ | Succinyl CoA+ Second CO2 + NADH | |
Succinyl CoA | Succinyl CoA synthetase/ succinate thiokinase | Cleaves | GDP | Succinate + GTP | Succinyl phosphate |
Succinate | Succinate dehydrogenase | Dehydrogenation | FAD | Fumarate + FADH2 | |
Fumarate | Fumarase | Hydration of double bond | OH- and H+ | Malate | Carbanion |
Malate | Malate dehydrogenase | Oxidation of hydroxyl group | NAD+ | Oxaloacetate+NADH |
Oxidative phosphorylation= Electron transport chain + chemiosmosis
- All aerobic organisms oxidize metabolic fuels like glucose to consume oxygen and generate carbon dioxide. That essentially is the process of respiration.
- The complete oxidation of glucose by molecular oxygen generates carbon-dioxide
C6H12O6 +6H2O→6CO2 +24H+ +24e
This reaction can be split into 2 reactions:
C6H12O6 +6H2O→6CO2 +24H+ +24e−
6 O2 + 24 H+ + 24 e− → 12 H2O
- The first half of the reaction is carried out by the enzymes of glycolysis and citric acid cycle
- The second half of the reaction involves the transfer of electrons from the reduced fuel to oxygen. This is called the Electron Transport Chain
- The 12 electron pairs after glucose oxidation are not directly transferred to oxygen. They are rather transferred to coenzymes NAD+ and FAD to form 10 NADH and 2 FADH2
- The electron pairs are then passed through the electron transport chain in the mitochondria creating a proton gradient which eventually generates ATP. This is chemiosmosis.
- Where exactly in mitochondria does the ETC take place? Mitochondria has two membranes. The outer membrane is permeable to almost all small molecules. The inner mitochondrial membrane is not permeable to small molecules. The only way they can enter the mitochondria is through specific transporters. The enzymes involved in ATP synthesis and, ETC are present in the inner mitochondrial membrane.
3 main events occur during an ETC:
- Deoxidation of NADH and FADH2 by transferring their electrons to other substances, so they can participate in other oxidation reactions
- The electrons participate in a series of oxidation and reduction reactions through different redox centers (consisting of four enzyme complexes)
- Coupling of the transfer of electrons to expulsion of protons from mitochondria. This produces a proton gradient across the inner mitochondrial membrane. This gradient converts ADP to ATP via oxidative phosphorylation
A few components in the inner mitochondrial membrane are very crucial.
- Firstly, the NADH produced in the cytosol must enter the mitochondrial ETC for oxidation. However, only the electrons from the NADH can be transported to the inner mitochondrial membrane through the malate aspartate shuttle
- Formation of FADH2: the glycerophosphate shuttle is responsible for the FADH2 formation. How does this works?
- Oxidation of NADH by dihydroxyacetone phosphate (catalyzed by 3 phosphoglycerol dehydrogenase) to give NAD+
- Electrons of the resulting 3 phosphoglycerol are transferred to flavoprotein dehydrogenase (present in the inner mitochondrial membrane) to give FADH2
- ADP-ATP translocator:
- Most of the ATP generated in the mitochondrial matrix is used in the cytosol. An ADP-ATP translator on the inner mitochondrial membrane helps in that. In exchange, it gets an ADP from the cytosol
- Phosphate carrier
- Synthesis of ATP requires ADP and Pi. The Pi must return to the mitochondrial membrane once the ATP is utilized in the cytosol. This requires a phosphate carrier
Steps in an ETC
- Entry of electrons: the electrons are funnelled into the inner mitochondrial membrane by dehydrogenases
- The electrons are accepted by Nicotinamide nucleotides (NAD+ or NADP+) or Flavin nucleotides (FMN/FAD).
- Nicotinamide-linked dehydrogenase generates NADH or NADPH along with a proton and an oxidized substrate. What exactly happens here? Dehydrogenase removes two hydrogen atoms from the substrate (reduction of substrate). One of the hydrogen is transferred to NAD+/NADP+ and the other H+ is released into the medium. NADH is involved in catabolic reactions and NADPH is involved in anabolic reactions
- Similar to Nicotinamide, Flavoproteins also accept either one electron ( to give semiquinone) or two electrons (FADH2 or FMNH2)
- Three ways in which electron transfer can take place
1. Direct transfer of electrons
2. Transfer of a hydrogen atom + 1 electron
3. Transfer of a hydride+ 2 electrons - In addition to NAD and FMN, three other electron acceptors or electron-carrying molecules are part of the ETC. They are: ubiquinone (CoQ), cytochromes, and iron-sulfur protein
- Ubiquinone can become semiquinone radical (QH) by accepting one electron and ubiquinol by accepting two electrons. This electron acceptor is very important in coupling the electron flow to proton movement (crucial for chemiosmosis) as it can carry both electrons and protons
- Cytochromes fall into three categories: a, b, and c
Order in which the electron pass through the ETC
The electrons passes through four enzyme complexes and finally reach oxygen to give H2O.
Let’s look at the four complexes
Complex 1: NADH to Ubiquinone (Q)
Also known as NADH dehydrogenase, it is a large enzyme with 42 different polypeptides including flavoproteins and 6 Fe-S centers. They carry out two functions:
- Transfer of a hydride from NADH to Q and a simultaneous transfer of protons from matrix to Inter membrane space (IMS).
NADH + H+ + Q= NAD+ + QH2
The QH2 diffuses into the inner mitochondrial membrane to reach complex 3 and oxidizes to Q - Transfer of four protons (H+) from matrix to IMS
Drugs like rotenone prevent the transfer of hydride from NADH to Q and thereby block oxidative phosphorylation.
Complex 2: Succinate to Ubiquinone
This complex consists of 5 prosthetic groups (2 types each) and 4 protein subunits
Subunits A&B has the following components:
- three 2Fe-2S centres
- FAD
- Succinate
- Subunits C&D has the following components:
- Heme b
- Binding site for Q
The order of electron transfer is
succinate——FAD——Fe-S centre——- Q binding site
Complex 3: Ubiquinone to cytochrome c
Also known as cytochrome bc-1 complex, it transfers electrons from QH2 to cytochrome c. In parallel, there is a vertical transport of protons from the matrix to IMS
At the end of electron transfer, QH2 is oxidized to Q, and 2 molecules of cytochrome c are reduced.
Complex 4:
Also known as cytochrome oxidase, it carries the electrons from cytochrome c to oxygen, which eventually reduces to H2O. It is a large enzyme with many subunits and components. The order of electron transfer is
Cyt c—— CuA——-Heme a——- Heme a3-CuB——- O2
For every 4 electrons through this complex, 4 H+ travel from matrix to IMS thereby reducing Oxygen to water.
Let’s look at the journey of electrons through the electron transport chain
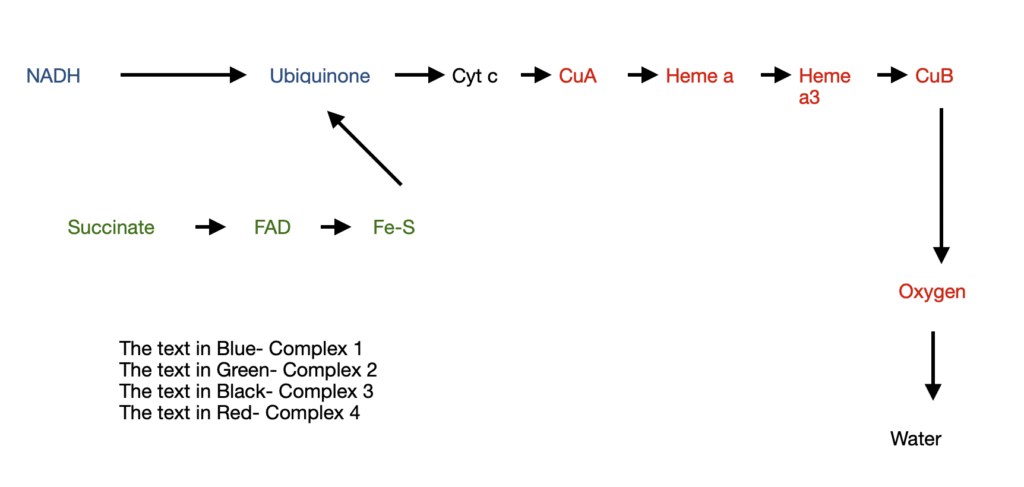
This entire electron transfer through these four complexes is primarily for one reason: to pump protons out of the matrix. For each pair of electrons being transferred,
4 protons are released from Complex 1
4 protons are released from Complex 3
2 protons are released from Complex 4
The energy stored in such a gradient is called the proton motive force (PMF). The PMF has two kinds of potential energy in it:
- Chemical potential energy: arising due to the difference in concentration of a chemical across the membrane
- Electric potential energy: arising due to separation of charge due to a proton moving to inter-membrane space with no counter ion
This PMF is what drives the next process in oxidative phosphorylation i.e. ATP synthesis
ATP synthesis
The basis for ATP synthesis is the chemiosmotic theory
ATP synthase, also known as complex 5 helps in ATP synthesis. It has two domains: F0 and F1
F0= peripheral membrane protein
F1= integral membrane protein
This enzyme catalyzes the formation of ATP from ADP and Pi along with the flow of protons from the matrix to IMS.
ADP + Pi——ATP synthase———— ATP
But, the ATP does not leave the surface of the enzyme until the proton gradient is established
So, the enzyme must alternate between a conformation that binds tightly to the ATP and the one that binds loosely to the ATP.
Each beta subunit of the ATP synthase exists in three different confirmations:
β ATP – very tightly bound to ATP
β ADP- loosely bound to ATP
β empty- no ATP. This is the conformation which releases the ATP from the enzyme
The basis for this is the binding change model.
At any given time, the active sites of F1 take turns catalyzing ATP synthesis. It starts with β ADP—-binds ADP + Pi—— β ATP—proton gradient—- β empty
These changes are due to the vertical movement of protons.
In addition to these reactions, gluconeogenesis i.e. the synthesis of glucose from non carbohydrate sources is very crucial. Check out my previous post for the same.
Very detailed and useful, thanks for sharing!